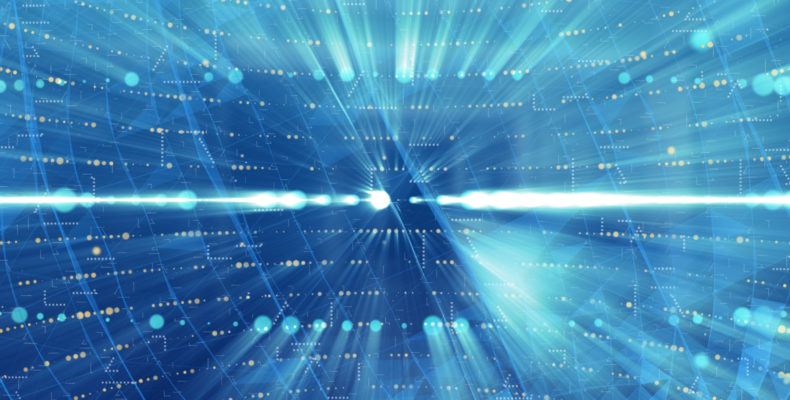
Going beyond graphene: the emergence of novel two-dimensional materials
Graphene was once described as a “rising star on the horizon of materials science”, and since then has been the subject of rigorous fundamental and applied research. Following its discovery in 2004, hundreds of companies worldwide have started producing graphene-based products. However, a major disadvantage is that pristine graphene lacks an energy bandgap, limiting its full usefulness in various applications.
Nonetheless, its discovery has spurred the investigation into a diverse range of alternative two-dimensional (2D) materials. One study indicated that there are as many as 1825 different known compounds that could potentially be used to isolate such 2D materials. One such family is known as transition metal dichalcogenides (TMDCs). Like graphene, these materials comprise an atomically thin sheet of a crystalline solid. Unlike graphene, however, monolayer TMDCs have an optical bandgap, and owing to their reduced dimensionality, possess fundamentally different properties compared to their bulk counterparts. These properties make them a highly attractive option for their integration into the next general electronic and optoelectronic devices.
Over the last decades, advancements in semiconductor electronics has driven ‘scaling’ of conventional silicon-based transistors (MOSFETs) to a point where they are soon expected to reach fundamental limits of their dimension. Here, TMDCs could offer a solution not only in terms of scalability, but also in terms of energy efficiency. Using one or more monolayers of TMDCs as a channel allows the reduction of transistor dimensions well below the limit of MOSFETs. Additionally, TMDCs such as monolayer molybdenum disulfide possess a large direct bandgap, which is not only essential for switching (a fundamental requirement of any transistor), but it can also be utilised to make an interband tunnel field-effect transistor that consumes less power than MOSFETs.
TMDCs could also pave the way for technologies beyond electronics. This is because several monolayer TMDCs have a honeycomb (or a hexagonal) lattice structure, which gives rise to two distinct ‘valleys’ in their electronic band structure. These valleys couple independently to specific circularly polarised light (right or left), opening up an additional degree of freedom that can be used to construct novel devices. For example, using this unique property, Y. J. Zhang et al. reported the world’s first demonstration of electrical control of circularly polarized light emission from several monolayers of tungsten diselenide, with potential implications in areas such as 3D displays.
Materials such as molybdenum disulfide and tungsten diselenide are also potential candidates for highly sensitive photodetectors for detection of light in the optical and near infrared region. Moreover, electrodes constructed from stacked monolayers of chemically exfoliated molybdenum disulfide have also been shown to exhibit high electrical conductivity and ion storage capability. This could have potential applications in the construction of the next generation high power and energy density supercapacitors and ion batteries.
One way to expand the range of properties that 2D materials can offer is to fit different monolayers together. For example, monolayers made from different compounds could be stacked vertically (heterostructure layers) or laterally (heterojunctions), with such structures exhibiting novel optical and electronic properties unlike those found in the constituent layers. Another way would be to chemically functionalise the surface of 2D materials with different molecules.
Moving forward, the challenge is to bring proof-of-concept prototype devices into the industrial arena by developing scalable and cost-effective ways to synthesise high-quality (defect free) 2D materials.
A critical step is to be able to synthesise high quality wafer-scales with unform thickness. Here, several techniques have shown promise, including chemical vapour deposition (CVD), which has been shown to produce high quality centimetre scale atomically thin layers of 2D materials. Optimisation of key parameters (for example, temperature and choice of substrates) during the CVD process will lead to further enhancements in the quality of 2D materials. Other techniques include, but are not limited to, pulsed laser deposition and thermal and plasma enhanced atomic layer deposition, both of which offer excellent thickness control and uniformity over a large area. However, these techniques are relatively complex, and processing costs remain high.
One solution would be to integrate these materials into the well-established silicon fabrication line. This could enable the production of high performance 2D material-based devices using silicon complementary Metal-Oxide Semiconductor (CMOS) logic, whilst keeping the production costs comparable to that of conventional silicon technology.
Yet, the full extent of the benefits that these materials can bring may not be realised if they are thought of simply as an addition to or a replacement of silicon. For progress to occur, a coordinated effort between the end-user companies, standardisation and validation institutes, academic labs and the like is required.
Somewhat unsurprisingly, the number of yearly patent filings in this field are still dominated by graphene. However, with an ever-growing number of alternative 2D materials at one’s disposal, patenting activity related to such materials is expected to pick up in the years to follow.
If you have a 2D material related invention you would like to protect, please do not hesitate to contact your usual Barker Brettell attorney.